6-Hydroxydopamine로 유도된 In Vitro 파킨슨병 모델에서 토란추출물의 Brain Resilience에 미치는 영향
Effects of Taro Extract on Brain Resilience in In Vitro Parkinson’s Disease Model Induced by 6-Hydroxydopamine
Article information
Abstract
Purpose: The purpose of this study was to investigate the effects of taro extract on brain resilience in in vitro Parkinson’s disease model induced by 6-hydroxydopamine (6-OHDA). Methods: To induce a neuroinflammatory reaction and the in vitro Parkinson’s disease model, SH-SY5Y cells were stimulated with lipopolysaccharide (LPS) and 6-OHDA, respectively. After that, cells were treated with at various concentrations (1, 5, and 10 mg/mL) of taro extract. Then nitric oxide (NO) production, inducible nitric oxide synthase (iNOS), interleukin (IL)-6, synaptophysin (SYP) and growth associated protein (GAP)-43 messenger ribonucleic acid (mRNA) expression level were measured. Results: Taro extract significantly suppressed LPS-induced NO production. Meanwhile, iNOS and IL-6 mRNA expression decreased in a dose-dependent manner. In addition, taro increased the mRNA expression of SYP and GAP-43 mRNA. Conclusion: These findings indicate that taro played an important role in brain resilience by inhibiting neuronal cell death and promoting neurite outgrowth, synaptogenesis, and neural plasticity. The results of this study suggest that taro may contribute to the prevention of neurodegenerative disease and become a new and safe therapeutic strategy for Parkinson’s disease.
서 론
1. 연구의 필요성
신경세포가 손상된 경우 세포사멸 또는 생존에 대한 경로 결정은 내인성(endogenous) 및 외인성(exogenous) 요인들의 활성화에 의존한다[1,2]. 신경세포 분화와 성장을 위한 신경영양인자와 전사인자 그리고 항산화인자 등은 대표적인 내인성 인자로 이들의 활성은 신경생성(neurogenesis), 신경세포분화(neural differentiation), 신경연 접생성(synaptogenesis) 및 신경 가소성(neural plasticity)을 강화시켜 신경회로(neural circuit)를 재구축함으로써 뇌는 손상 이전의 상태로 복구되고자 하는데 이러한 뇌의 회복능력(brain resilience)은 건강한 노화와 질병예방을 위해 매우 중요하다[1,3-5].
파킨슨병은 유전적, 환경적 요인으로 인해 미토콘드리아 기능장애, 세포산화반응으로 인한 DNA (deoxyribonucleic acid), alpha-synuclein의 응집 등으로 도파민성 신경세포가 사멸되어 진전(tremor), 강직(rigidity), 운동완서(bradykinesia)와 같은 운동장애 및 변비, 우울, 수면장애, 기억장애와 같은 비운동장애가 동반되어 나타나는 만성신경퇴행성 질환이다[6,7]. 우리나라는 노인 인구가 증가함에 따라 파킨슨병 유병율도 증가하고 있는데[8], 최근 일부 선행연구에서 다제약제(polypharmacy)가 노년기 파킨슨병 발병의 위험을 높이고 있으며 특히, 노인 한명 당 복용하는 약물 수가 많을수록 파킨슨병 위험이 증가하였음을 보고하였다[9,10]. 노인인구의 증가는 이와 같은 질병 및 건강문제로부터 안전할 수 있는 방법과 건강한 노화로 이행되는 것에 대한 사회적 관심을 증폭시켰으며 그 결과 녹차, 허브, 홍삼, 인삼, 커큐민(curcumin), 오메가 3, 버섯, 감국 및 토란 등의 다양한 천연물소재(natural products)가 개발되고 소비되고 있다[11]. 건강기능식품 형태로 가공되어 음용하고 있는 대부분의 천연물 소재는 면역기능 활성, 비만억제, 항염증 및 항산화에 대한 기능과 당뇨와 암 등의 질병진행 억제에 효과가 있는 것으로 나타났는데, 이와 같은 천연물소재는 안전하고 부작용이 적어 건강예방활동을 위한 가장 보편적인 방법으로 활용할 수 있다는 장점이 있다[12-14]. 따라서 본 연구에서는 만성신경퇴행성질환 대상자들의 질병예방 및 건강증진활동을 위한 천연물소재 활용의 과학적 근거를 제시하고 이를 통해 다제약제 발생 감소 및 노년기 삶의 질 개선에 대한 방안을 모색하고자 한다.
토란(Colocasiae sculenta, Taro)은 천남성과(Araceae family)에 속하는 구근식물(tuberous plants)로 잎, 줄기, 알줄기 등 식물의 모든 부분이 식용가능하나 땅속의 알줄기 부분을 가장 많이 섭취하며 탄수화물, 단백질, 필수아미노산, 칼륨, 식이섬유소 및 비타민 C 함유량이 높고, 수분용해성이 높아 식품에서의 활용도가 매우 좋다[15,16]. 토란은 우리나라에서도 널리 재배되어 구입이 쉽고, 비용도 저렴하며 실제로 식생활에서 다양한 조리형태로 섭취하고 있어 천연물소재로서 활용도가 높다고 할 수 있다. 다만 생토란을 섭취할 경우 옥살산칼슘(calcium oxalate)의 독성 작용으로 인해 복통, 신장결석 등의 부작용이 발생할 수 있으나 열처리나 조리과정을 통해 감소시킬 수 있다[15,17]. 토란의 영양학적 특성은 식물전체에서 유사한 분포를 보이나 특히, 알줄기에는 flavonoids, alkaloids, steroids, β-sitosterol, tarin, lectin 등과 같이 생물학적 활성을 갖는 성분들이 풍부한 것으로 알려져 있는데[16] 선행연구에서 토란의 β-sitosterol은 지방친화성 물질로 혈액-뇌 장벽(blood–brain barrier)을 통과할 수 있어 신경약리학적 기능이 가능함을 제시하였다[12].
최근 일부연구에서도 토란, 측백엽 추출물 및 홍삼 등이 각각 강박장애 동물모델의 행동 개선과 도파민성 신경세포 사멸억제에 대한 효과가 있는 것으로 나타나[12,18,19], 천연물소재가 신경계 질환에서도 예방 및 치료적 전략이 될 수 있을 것으로 생각한다. 선행연구에서 토란은 혈당과 혈중 지질 개선 및 고혈압, 당뇨, 비만 등의 위험을 감소시키며 항산화 및 항염증 효과가 있는 것으로 나타났으나[15,20,21], 파킨슨병과 알츠하이머병과 같이 나이가 들수록 유병률이 증가하는 신경퇴행성 질환에서 토란의 효능을 확인한 연구는 거의 없었다. 최근 파킨슨병에서 도파민성 신경세포의 사멸은 손상으로 인한 별아교세포(astrocyte) 및 미세아교세포(microglia)와 같은 신경아교세포(neuroglia)의 활성으로 촉발된 신경염증(neuroinflammation) 반응의 결과라는 보고가 있었다[22-24]. 또한 in vitro 파킨슨병 모델에서 축삭손상 및 퇴행이 신경세포의 세포체(cell body) 손상에 선행되어 나타나므로 축삭퇴행 경로를 차단하고 축삭의 재성장을 촉진함으로써 신경세포를 생존으로 유도할 수 있다는 가능성을 제시하였다[1,5].
따라서, 본 연구에서는 인간 유래 신경아세포종(human neuroblastoma)이며 도파민성 신경세포 주의 하나인 SH-SY5Y 세포에 그람음성균의 내독소(endotoxin)인 LPS (lipopolysaccharides)를 처리하여 신경염증을 유발한 후 토란추출물을 처치하여 토란의 신경염증 반응 억제효과를 확인하고자 한다. 또한, 도파민 수용체에 대한 높은 친화력으로 도파민성 신경세포에 대한 독성효과를 가진 6-hydroxydopamine (6-OHDA)를 처리하여 in vitro 파킨슨병 모델을 유발한 후 토란추출물을 처치하여 신경돌기성장(neurite outgrowth), 신경연접생성 및 신경 가소성에 대한 토란의 기능을 확인하고자 한다. 이를 통해 본 연구에서는 손상으로 인한 신경계 변화로부터 신경세포의 생존을 유도하는 뇌의 회복능력에 토란이 미치는 영향을 규명하고자 하며 본 연구결과는 토란추출물의 파킨슨병의 예방과 표적치료를 위한 기초자료로 활용할 수 있을 것으로 기대한다.
연구 방법
1. 시약 및 기기
Fetal bovine serum (FBS), 항생제(penicillin/streptomycin), Dulbecco’s modified Eagle medium (DMEM), Trypsin-EDTA (ethylenediaminetetraacetic acid) 등의 세포 배양용 시약들은 Life Technologies (Grand Island, NY, USA)에서 구입하였으며, in vitro 파킨슨병 모델 유 도를 위한 6-OHDA 그리고 세포독성 및 유전자 발현 검증을 위한 LPS, crystal violet, formalin, chloroform, isopropanol, Tri-reagent (Trizol)은 Sigma-Aldrich Corporation (St. Louis, USA)에서 구입하여 사용하였다.
2. 실험재료 및 토란 추출
본 실험에서 사용한 토란은 경기도 이천시 마장면에서 공급된 알토란으로 지역 농협에서 구입하여 사용하였다. 토란은 물로 씻어 지름 1-3 cm, 두께 1 cm 정도의 크기로 세절한 후, 동결건조 하여 토란 내 수분을 제거하였으며, 이후 분쇄기(HR-2860, Philips Korea, Seoul, Korea)를 이용하여 분말화 하였다. 분말화된 토란 30 g에 distilled water (D.W) 800 mL을 첨가하여 40°C에서 24시간 동안 저온 추출한 후 불순물 제거를 위해 감압여과를 진행하였으며 여과된 토란 물 추출물은 추가 동결 건조를 실시함으로써 수분이 모두 날아간 분말 형태의 최종 토란 물 추출물을 얻어내었다. 실험에 사용할 토란추출물은 4°C에 냉장 보관한 후 D.W에 녹여 1, 5&10 mg/mL 농도로 준비하여 실험에 사용하였다.
3. 세포배양
인간 유래 신경아세포종인 SH-SY5Y 세포는 한국세포주은행(Korean Cell Line Bank, Seoul, Korea)에서 분양받아 실험에 사용하였으며, 10% FBS와 1% penicillin/streptomycin 100 unit/mL가 첨가된 DMEM 배지를 사용하여 5%CO2, 37°C 조건하에서 배양하였다.
4. 세포생존율 및 Nitric Oxide (NO) 생성량 측정
토란의 SH-SY5Y 세포에 대한 독성을 검증하기 위해 crystal violet assay를 통해 세포생존율을 확인하였다. SH-SY5Y 세포를 24 well plate의 각 well 당 5×105개가 되도록 분주하여 24시간 동안 배양한 후 6-OHDA (100 μM)와 토란추출물을 1, 5&10 mg/mL 농도로 처리한 다음 24시간 동안 추가 배양하였다. 그 후 배양액을 제거하고 phosphate buffered saline (PBS)로 세척한 다음 10% formalin 용액으로 실온에서 10분간 고정시켰으며 다시 PBS로 세척한 후 1% crystal violet 용액으로 세포를 10분간 염색하였다. 이후 증류수로 염색 용액을 세척한 후 물기를 건조시켰다. 마지막으로 세포에 염색된 crystal violet은 33% acetic acid를 사용하여 녹인 뒤 용액을 96 well plate에 옮겨 microplate reader (Molecular Devices, CA, USA)를 이용하여 595 nm에서 흡광도를 측정하였다. NO 생성량은 Nitrite assay kit (BioVision, CA, USA)을 이용해 세포 배양액 내 존재하는 NO의 안정된 산화물인 NO2-를 측정하여 분석하였다. SH-SY5Y 세포를 24 well plate에 1×106 cells/well의 농도로 분주한 후 24시간 배양한 뒤 배지를 버리고 PBS로 세척한 다음 FBS를 포함하지 않는 새로운 배지로 교환하였다. 그 후 LPS (1 μg/mL)와 토란추출물을 농도별로 처리하여 24시간 추가 배양한 뒤 상층액을 얻은 후, 상층액 100 μL와 assay 시약 100 μL을 혼합하여 96 well plate에서 10 min 동안 반응시킨 다음 microplate reader를 이용해 540 nm에서 흡광도를 측정하였다.
5. Neurite outgrowth assay
SH-SY5Y 세포에 6-OHDA를 처리한 후 토란추출물에 의한 신경돌기 성장의 변화를 확인하기 위해 신경돌기 길이를 현미경(Olympus BX40; Olympus, Tokyo, Japan) 200배 하에서 사진을 촬영하여 측정하였다. 각각의 그룹별로 5개의 개별 구역(discrete field)을 확보하고, 각 시야에서 50개 이상의 SH-SY5Y 세포의 신경돌기의 길이를 측정한 후 OptiView 4.10 (KOREALABTECH, Seongnam, Gyeonggi-do) 프로그램을 이용하여 분석하였다.
6. Reverse Transcription Polymerase Chain Reaction (RT-PCR)
토란의 신경염증에 대한 기능을 검증하기 위해 inducible nitric oxide synthase (iNOS)와 interleukin (IL)-6의 messenger ribonucleic acid (mRNA) 발현 정도를 RT-PCR 방법으로 분석하였다. SH-SY5Y 세포를 24-well plate (1×106 cells/well)에 분주한 후 24시간 동안 배양한 다음 농도별 토란추출물과 LPS (1 μg/mL)를 동시 처리하여 24시간 추가 배양하였다. 이후 세포를 거둬 PBS로 세척한 다음 Trizol을 넣어 세포를 용해시킨 후 chloroform을 추가하여 13,000 rpm에서 15분간 원심 분리하여 상층액을 얻었으며 이후 상층액과 동량의 isopropanol을 섞은 후 4°C에서 15분간 침전시킨 후 total RNA을 분리하였다. 분리한 Total RNA를 oligo (dT) 18 Primer, deoxynucleotide triphosphate (dNTP) 0.5 μM, 1 unit ribonuclease (RNase) inhibitor 그리고 Superscript II reverse transcriptase가 포함된 AccuPower® RT-PCR PreMix kit(Bioneer, Daejeon, Korea)를 이용하여 42°C에서 60 min, 그리고 94°C에서 5분간 반응하여 1 μg의 complementary deoxyribonucleic acid (cDNA)를 합성하였다. 합성된 cDNA와 iNOS, IL-6 및 beta-actin (β-actin) primer를 AccuPower® RT-PCR PreMix kit에 혼합하여 제조사의 지침에 따라 94°C에서 30초, 58°C에서 30초, 72°C에서 1분의 조건으로 총 35 cycles로 Thermal Block (AllinOneCycler, Bioneer, Daejeon, Korea)을 이용하여 RT-PCR 수행하였다. PCR에 의해 생성된 산물은 1.2% agarose gel에서 전기영동을 실시하였으며 Neogreen (CELLGENTEK, Cheongju, Korea)으로 염색한 후 Geldoc (FluoroBox, CELLGENTEK, Cheongju, Korea)을 이용해 band를 확인하였다. 각 mRNA band의 강도에 대한 정량적 비교를 위하여 housekeeping 유전자인 β-actin으로 표준화하였으며 분석에 사용된 각각의 primer 염기서열은 Table 1에 나타내었다.
7. Real Time PCR
토란의 신경연접생성과 신경 가소성에 대한 기능을 검증하기 위해 synaptophysin (SYP)과 growth associated protein (GAP)-43의 mRNA 발현 정도를 real time PCR 방법으로 분석하였다. SH-SY5Y 세포를 6-well plate (3×104 cells/well)에 분주한 후 24시간 동안 배양한 다음 농도별 토란추출물과 6-OHDA (100 μM)를 동시 처리한 후 24시간 추가 배양하였다. 이후 세포를 거둬 PBS로 세척한 후 Trizol을 이용하여 total RNA를 분리하였다. 분리한 Total RNA로부터 AccuPower® RT-PCR PreMix kit을 이용하여 제조사의 지침에 따라 1 μg의 cDNA를 합성하였다. 합성된 cDNA, SYBR Green Supermix (Bio-rad, CA USA), 각각의 sense, antisense primer (Bioneer, Daejeon, Korea)를 혼합하여 95°C에서 10분간 반응시킨 후 95°C에서 30초, 58°C에서 30초의 조건으로 40 cycles로 real time PCR (CFX 96 Real-Time PCR Detection System, Bio-Rad, CA, USA)을 진행하였다. 증폭이 역치에 도달한 Ct (threshold cycle) 값을 기준으로 standard curve를 그려서 정량화하였으며 SYP&GAP-43의 mRNA Ct 값을 β-actin mRNA Ct 값으로 보정하였다. 분석에 사용된 각각의 primer 염기서열은 Table 1에 나타내었다.
8. 통계분석
모든 실험은 3회 이상 반복 수행하였으며 결과는 SPSS WIN 23.0 프로그램을 이용하여 평균과 표준편차를 계산하여 그래프로 나타내었다. 또한, 각 변수는 Kruskal-Wallis를 사용하여 비모수 검정을 하였으며 사후검정은 Mann-Whitney U test with bonferroni correction을 이용하였다. 모든 통계량의 유의수준은 p<.05에서 채택하였다.
연구 결과
1. 6-OHDA 세포독성에 대한 토란추출물의 세포 생존력 및 NO 생성에 미치는 영향
6-OHDA로 유도된 세포독성에서 토란추출물이 세포 생존에 미치는 영향을 확인하기 위해 세포생존율을 측정한 결과 토란추출물은 6-OHDA 단독 처리군에 비해 모든 농도에서 세포에 대한 독성이 나타나지 않았으며(χ2=27.95, p<.001) 농도 의존적으로 유의하게 세포 생존율이 증가하였다(1, 5&10 mg/mL, U=0.00, p<.01)(Figure 1A). 또한 LPS로 유도된 NO 생성을 측정한 결과 토란추출물을 처리하였을 때 LPS 단독 처리군에 비해 모든 농도에서 NO 생성이 감소하였으며(χ2=26.11, p<.001), 1 mg (U=0.00, p=.015), 5&10 mg/mL (U=0.00, p=.003) 각각 농도 의존적으로 NO생성이 유의하게 감소하였다(Figure 1B).

Effect of taro extract on 6-OHDA-induced cell viability (1-A) and LPS-induced nitric oxide (1-B) in SH-SY5Y cell. There was no cytotoxicity on SH-SY5Y cell with taro extract up to 10 mg/mL. Taro extract significantly reduced the LPS-stimulated nitrite production compared with LPS alone treated group in a dose-dependent manner. *p<.05, **p<.01 compared to 6-OHDA&LPS stimulation. 6-OHDA=6-hydroxydopamine; LPS=Lipopolysaccharides.
2. 토란추출물의 신경염증 및 세포사멸 조절 관련 mRNA 발현에 미치는 영향
LPS를 SH-SY5Y 세포에 처리하여 염증반응을 유발한 후 iNOS와 IL-6 mRNA 발현을 확인하였다(Figure 2A). 그 결과 토란추출물은 모든 농도에서 iNOS (χ2=20.66, p<.001)와 IL-6 (χ2=26.12, p<.001) mRNA 발현을 감소시키는 것으로 나타났으며 LPS를 단독처리한 군에 비해 모든 농도에서 통계적으로 유의하게 iNOS와 IL-6 mRNA 발현을 억제하는 것으로 나타났다(1, 5&10 mg/mL, U=0.00, p=.004)(Figure 2B, C).
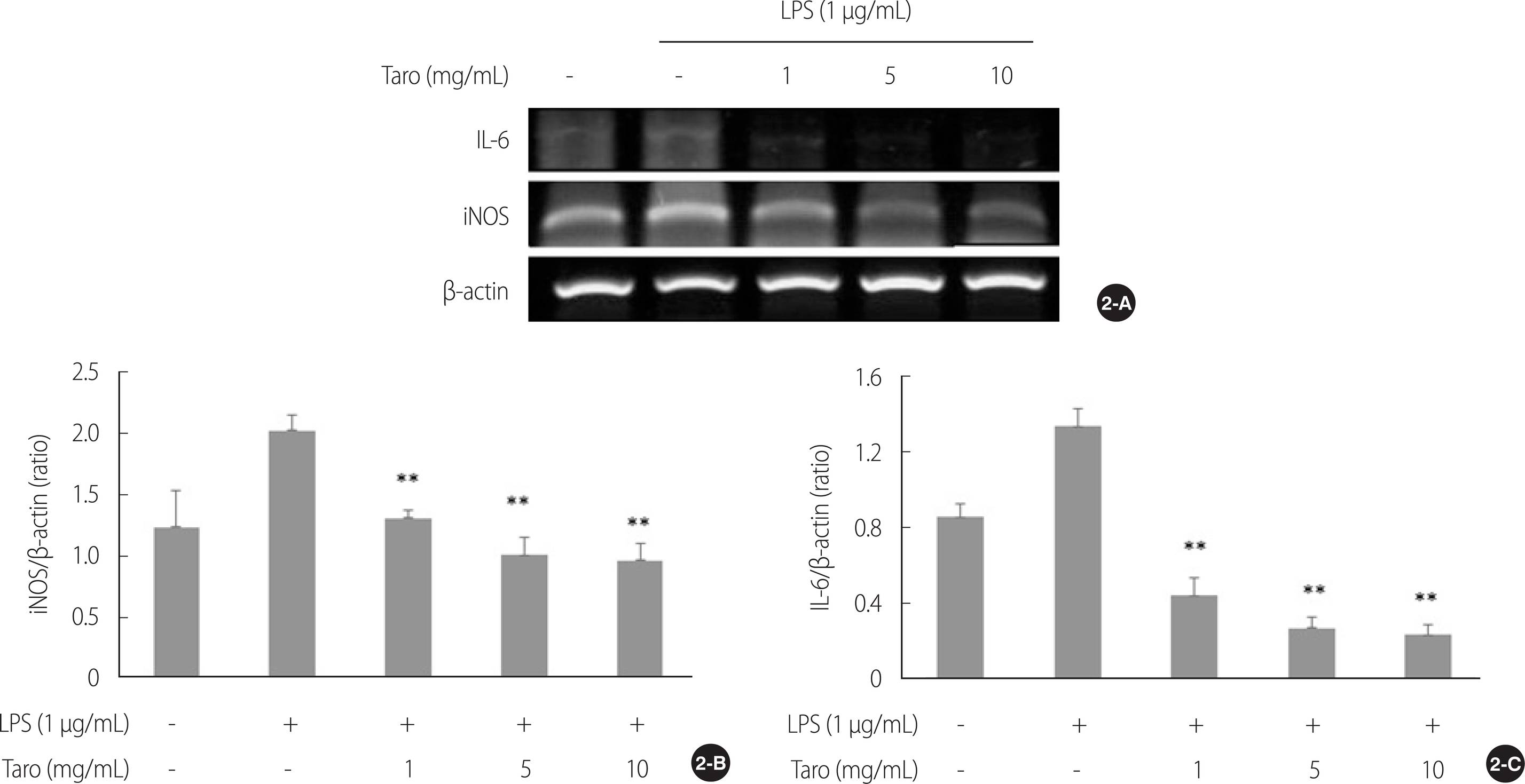
Effect of taro extract on LPS-induced mRNA expression in SH-SY5Y cell. The iNOS and IL-6 mRNA expressions were analyzed by RT-PCR (2-A). All gene expressions were normalized using β-actin as a reference gene. The iNOS (2-B) and IL-6 (2-C) mRNA expressions were significantly decreased in taro extract treated group compared with LPS treated group. **p<.01 compared to LPS stimulation. LPS=Lipopolysaccharides; iNOS=Inducible nitric oxide synthase; IL-6=Interleukin-6; β-actin=beta-actin; RT-PCR=Reverse transcription polymerase chain reaction.
3. 토란 추출물의 신경돌기 성장에 미치는 영향
6-OHDA로 유도된 in vitro 파킨슨병 모델에서 토란추출물에 의한 신경돌기 성장의 변화를 확인한 결과 6-OHDA를 단독 처리한 군에 비해 토란추출물을 처리한 모든 군에서 신경돌기 성장 및 주변 세포와의 연결이 증가하는 양상을 보였다(Figure 3A). 또한, SH-SY5Y 세포 신경돌기의 길이를 측정한 결과(χ2=27.21, p<.001), 6-OHDA를 단독 처리한 군에 비해 모든 토란추출물 농도에서 신경돌기의 길이가 통계적으로 유의하게 증가하는 것으로 나타났다(U=0.00, p=.004)(Figure 3B).
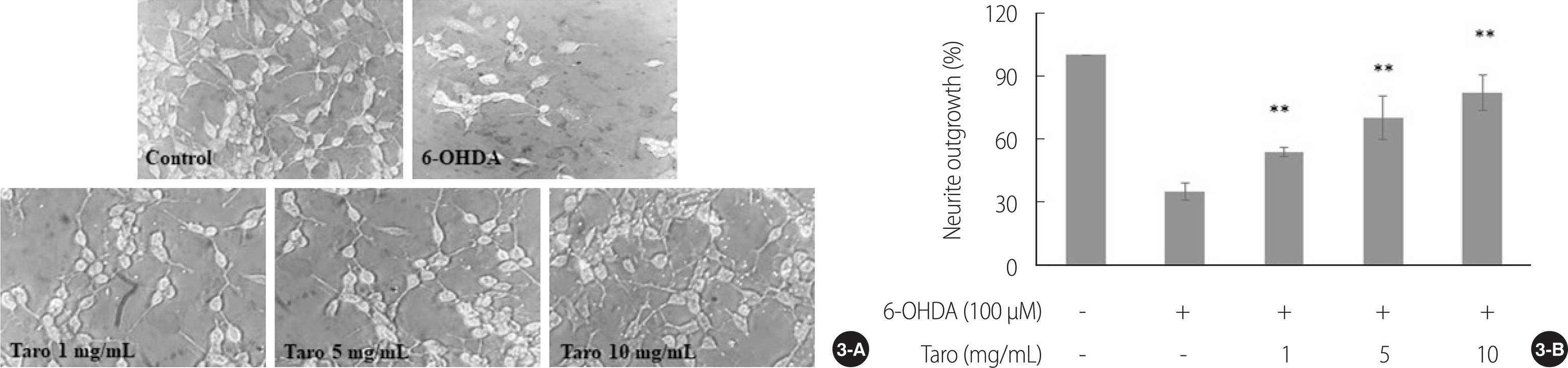
Neurite outgrowth&neuronal differentiation were visualized under×200 magnifications. Taro stimulated neurite outgrowth in SH-SY5Y cell (3-A). The length of dendrites of SH-SY5Y cell was significantly increased in taro extract treated group compared with 6-OHDA alone treated group in a dose-dependent manner (3-B). 6-OHDA=6-hydroxydopamine. *p<.05, **p<.01 compared to 6-OHDA stimulation.
4. 토란추출물의 신경연접생성과 신경 가소성 관련 mRNA 발현에 미치는 영향
토란추출물의 신경연접생성과 신경 가소성에 미치는 영향을 확인하기 위해 SYP와 GAP-43 mRNA 발현을 real time PCR로 확인하였다(Figure 4A). 신경세포의 시냅스 표지자인 SYP mRNA 발현을 확인한 결과(χ2=16.11, p=.003), 6-OHDA를 단독 처리한 군에 비해 5 & 10 mg/mL 농도에서 통계적으로 유의하게 mRNA 발현이 증가하였다(U=4.00, p=.025)(Figure 4B). 한편, 축삭성장과 재생에 관여하는 GAP-43 mRNA 발현을 확인한 결과(χ2=25.36, p<.001), 6-OHDA를 단독 처리한 군에 비해 모든 토란추출물 농도에서(U=0.00, p=.004) mRNA의 발현이 유의하게 증가하였음을 확인하였다(Figure 4C).

Effect of taro extract on SYP and GAP-43 mRNA expression in SH-SY5Y cell. The SYP and GAP-43 mRNA expression were analyzed by real time PCR (4-A). All gene expressions were normalized using β-actin as a reference gene. The SYP mRNA expression were significantly increased in all taro extract treated groups except 1mg/ml, compared with 6-OHDA alone treated group (4-B). Meanwhile, GAP-43 mRNA expression were significantly increased in taro extract treated group compared with 6-OHDA alone treated group in a dose-dependent manner (4-C). *p<.05, **p<.01 compared to 6-OHDA stimulation. 6-OHDA=6-hydroxydopamine; GAP-43=Growth associated protein-43; SYP=Synaptophysin; β-actin=beta-actin; PCR=Polymerase chain reaction.
논 의
본 연구에서는 SH-SY5Y 세포에 LPS와 6-OHDA를 처리하여 신경염증 및 in vitro 파킨슨병 모델을 유발한 후, 토란추출물을 처치함으로써 신경돌기성장, 신경연접생성 및 신경가소성에 대한 토란의 기능을 규명하고자 하였다. 본 연구에서 SH-SY5Y 세포에 LPS를 처리하여 신경염증 반응을 유도한 결과, iNOS와 IL-6의 mRNA 발현이 증가하였으며 토란의 처치로 인해 이들 발현이 감소한 것으로 나타났다. 선행연구에서 LPS 자극은 신경세포와 신경아교세포로부터 iNOS, IL-1, IL-6, IL-8 및 tumor necrosis factor (TNF)와 같은 pro-inflammatory 사이토카인의 생산을 유도하여 중추 및 말초신경조직의 염증을 유발하고 혈액 뇌 장벽을 손상시킨다고 하였다[2]. 특히, iNOS는 손상이나 염증성 사이토카인의 활성에 의해 다량의 NO를 생산하는데[25], 파킨슨병 환자의 사후 뇌 조직에서 상당한 양의 iNOS가 흑질(SNpc)에서 발견된 것으로 나타나 도파민성 신경세포의 퇴행에 NO가 깊이 관여하고 있음을 알 수 있다[26].
본 연구에서도 LPS를 단독 처리한 군에서 NO 생성이 증가하였다가 토란에 의해 감소되었으며 또한, 6-OHDA에 의해 증가한 iNOS 및 IL-6 mRNA 발현이 토란추출물을 처치한 군에서는 농도 의존적으로 감소하는 것으로 나타나 토란이 NO 생성 및 신경염증 반응에 관여하는 mRNA 발현을 조절하고 있음을 확인하였다. 선행연구에서 토란이 cyclooxygenase (COX)의 활성과 prostaglandins E2 (PGE2) 합성을 억제하여 항염증 및 항암 활성 등의 약리학적 작용을 하고 있음을 보고하였는데[16], 본 연구결과를 통해 토란이 신경염증 반응에도 관여함으로써 신경세포 퇴행 및 신경조직 손상 예방 역할을 할 수 있다는 가능성을 제시하였다.
신경세포는 증식하고 분화한 후 성장 및 성숙하게 되며 시냅스 형성을 위한 가지돌기와 축삭의 성장을 도모하는데 neuronal nuclei (NeuN), 신경영양인자(neurotrophic factors), GAP-43 및 SYP와 같은 특정 neuronal marker 등의 방출로 이 과정이 촉발되고 이때 형성된 시냅스는 유기체의 수명 동안 함께 지속된다[5,27]. 신경세포의 분화와 성장은 신경전달물질, 호르몬 등과 같은 분자들의 세포 내 이동으로 시작되어 형태학적, 기능적 변화의 통합과정을 통해 완성되는데[28,29], 본 연구에서는 SH-SY5Y 세포의 신경돌기 길이를 측정하여 토란이 신경돌기 성장에 미치는 영향을 확인하였다. 신경돌기 길이를 측정한 결과 6-OHDA를 단독처리한 군에 비해 모든 토란추출물 농도에서 신경돌기의 길이가 증가하였으며 주변 세포들과의 연결도 개선된 것으로 나타나 토란이 손상으로 인한 신경세포의 신경돌기 성장을 촉진하고 주변 신경세포와의 연접을 자극함으로써 신경회로의 정상적인 기능회복에 기여하고 있음을 확인하였다. 이는 신경돌기 성장과 시냅스 형성에 관여하는 유전자 발현을 통해서도 확인할 수 있었는데 GAP-43와 SYP mRNA 발현이 5 mg과 10 mg/mL와 같은 고농도의 토란추출물을 처리한 군에서 공통적으로 증가하는 것으로 나타났다. SYP는 뇌와 척수 등의 대부분의 중추신경세포에서 발현되는 presynaptic marker로 시냅스 전달과 시냅스 소포 주기에 중요한 기능을 하는데 선행연구에서 SYP의 부족은 학습 및 기억장애와 공간학습 능력을 감소시키며[30,31], GAP-43는 축삭과 시냅스 전 신경세포 말단의 중요한 구성 요소로 신경돌기 형성 및 재생에 관여하는 것으로 나타났다[5]. GAP-43는 mRNA나 단백질의 상승 여부와 관계없이 신경돌기 발달과 성장 그리고 신경가소성에 관여하며 특히, 손상 후 발현이 증가하여 hippocampus에서의 축삭 재생을 자극함으로써 기억강화를 촉진하는 것으로 나타났다[32].
손상된 신경세포의 생존을 위해서는 세포체뿐만 아니라 신경돌기가 구조적, 기능적 정상을 유지하며 표적세포와 신경연접이 지속적으로 유지되어야 하는데, 이처럼 정상적인 신경돌기의 존재는 파킨슨병이나 알츠하이머병과 같은 신경퇴행성질환에서도 세포퇴행이나 사멸을 지연시켜 뇌가 기능적으로 회복하는 데 중요한 기능을 한다[33,34].
본 연구의 결과에서 나타난 GAP-43 mRNA 발현의 변화가 6-OHDA에 의한 손상으로부터 회복하는 과정에서 GAP-43 mRNA가 과다 발현한 것인지 손상된 신경세포의 복구 과정을 토란이 촉발한 결과인지는 불분명하나 신경세포가 손상된 후 신경돌기 및 축삭의 재생과 신경가소성을 촉진하기 위해 GAP-43 mRNA 발현이 증가하였다는 선행연구[5,32]와 비교해 볼 때 토란이 GAP-43와 SYP mRNA 발현에 영향을 줌으로써 신경망의 재구성을 촉진하고 신경계의 기능적 회복을 유도할 수 있다는 가능성을 확인하였다. 선행연구에서 신경세포 간의 시냅스 손실은 뇌의 특정 영역의 기능 손상을 일으킬 뿐만 아니라 과도한 시냅스 손실은 알츠하이머병에서 인지기능장애 증상의 발현과 관계가 있으며[35], MPTP (1-methyl-4-phenyl-1,2,3,6-tetrahydropyridine)를 이용한 in vivo 실험에서 중뇌 흑질의 도파민성 신경세포체가 손실되기 전에 도파민성 신경세포의 축삭 손실이 선행된다고 하였다[36].
따라서, 토란이 SYP 및 GAP-43 mRNA 발현을 조절하고 있음을 밝힌 본 연구 결과는 신경손상에 대한 표적치료 및 퇴행성 증상을 개선하기 위한 새로운 치료적 전략으로 활용할 수 있을 것으로 기대한다. 본 연구에서는 신경퇴행성질환에서 토란이 신경염증 반응과 신경세포 생존에 관여하는 mRNA 발현을 조절함으로써 뇌 손상 후 회복능력에 기여하고 있음을 확인하였다. 그러나 본 연구에서 토란이 도파민성 신경세포 사멸을 억제하고, 생존을 유도하는 관련 인자들의 down signaling 경로 분석이 이루어지지 못한 점과 SH-SY5Y 세포 외에 다른 신경세포에서 토란의 작용을 확인하여 비교 분석하지 못한 점은 제한적이다.
결 론
본 연구에서는 손상으로 인한 신경계 변화로부터 신경세포의 생존을 유도하는 뇌의 회복능력에 토란이 미치는 영향을 확인하고자 하였다. 그 결과 6-OHDA로 유도된 in vitro 파킨슨병 모델에서 토란은 신경염증 반응을 억제하고 신경돌기성장, 신경연접생성 및 신경 가소성에 관여하는 내인성 인자의 발현을 촉진하는 데 기여하고 있음을 확인하였다. 본 연구는 토란의 도파민성 신경세포 보호 및 신경염증 조절과 신경돌기 재성장에 대한 효과를 규명하는 첫 번째 시도로 파킨슨병의 예방과 표적 치료를 위한 토란의 활용 가능성을 제시하였다는 점에서 의의가 있다. 또한, 본 연구결과는 질병 예방과 건강증진을 위한 천연물소재의 안전하고 올바른 사용에 대한 간호교육자료로 활용할 수 있을 것으로 생각한다. 본 연구결과를 토대로 in vivo 파킨슨병 모델을 유발하여 도파민의 생성과 투사가 일어나는 nigrostriatal 경로에 토란이 미치는 영향을 확인하는 중개 연구와 토란의 장기간 섭취로 인한 부작용 및 치료약물과의 약물 상호작용 등의 후속 연구를 통해 천연물소재를 안전하게 음용할 수 있는 방안에 대한 중재연구가 필요함을 제언한다.
CONFLICT OF INTEREST
The authors declared no conflict of interest.
AUTHORSHIP
CHY and KKA contributed to the conception and design of this study and performed the collecting data, the statistical analysis and interpretation; drafted the manuscript and critically revised the manuscript; KKA supervised the whole study process. All authors read and approved the final manuscript.